Construction and Working of Solar Cells
Know the details about the Construction and Working of Solar Cells, A solar cell, also known as a photovoltaic cell, converts sunlight directly into electricity through the photovoltaic effect. Typically made from silicon, these cells consist of a p-n junction, created by doping silicon with specific impurities.
When sunlight strikes the cell, photons energize electrons, knocking them loose and generating a flow of electric current. This process occurs in layers: the top layer absorbs light, while the bottom layer collects the free electrons. An anti-reflective coating enhances efficiency by minimizing light loss. The cells are then assembled into panels, which can be integrated into various applications, from residential rooftops to large-scale solar farms.
Table of Contents
Construction and Working of Solar Cells: A Comprehensive Guide
Introduction to Solar Cells
Solar energy is a renewable, abundant, and sustainable resource that has emerged as a pivotal solution to the energy crisis and environmental challenges of our time. Solar cells, also known as photovoltaic (PV) cells, are devices that convert to sunlight directly into electricity. Their use in power generation is not only environmentally friendly but also cost-effective in the long run. This blog post dives into the detailed construction, types, and working principles of solar cells, with a focus on how they are designed to harness the sun’s energy.
Basics of Photovoltaic Technology
Photovoltaic technology is the science behind converting sunlight into electricity. Solar cells are made from semiconducting materials that exhibit the photovoltaic effect. When sunlight hits the material, it excites electrons, which in turn generate electricity. This process is clean, renewable, and requires no fuel or emissions. The energy generated by solar cells can be used in various applications, ranging from powering homes and industries to fueling cars and spacecraft.
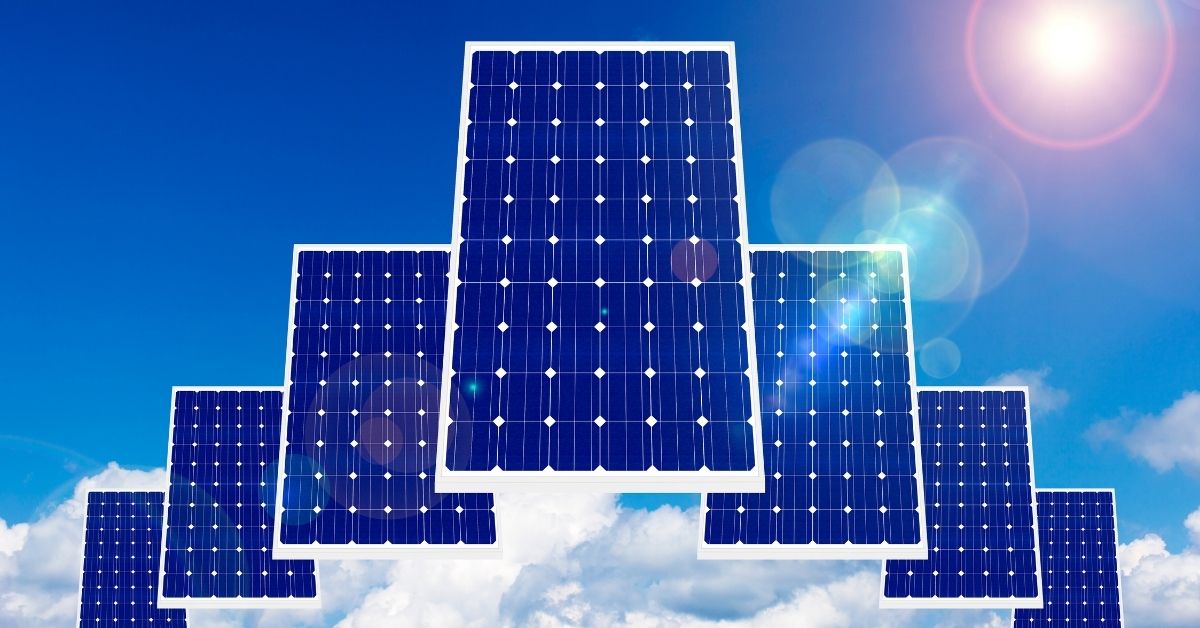
Key Components of a Solar Cell
A solar cell consists of several essential components, each playing a crucial role in the conversion of sunlight into electrical energy. These components include:
1. Semiconductor Material (Usually Silicon):
This is the core material where the photovoltaic effect occurs. Silicon, particularly crystalline silicon, is the most widely used semiconductor material in solar cells due to its abundance and favorable properties.
2. Front Contact:
The front contact, typically made of a thin metal grid, allows the electrons generated by the solar cell to flow into an external circuit. It must be transparent enough to allow sunlight to pass through but conductive enough to collect the current.
3. Anti-Reflective Coating:
Since solar cells need to absorb as much sunlight as possible, an anti-reflective coating is applied to reduce the amount of light reflected away from the cell. This increases the efficiency of the cell by ensuring more photons are absorbed.
4. Back Contact:
The back contact is a metal layer at the bottom of the solar cell. It helps collect the current and transmit it to an external circuit, completing the electrical pathway.
5. Glass Covering or Encapsulation:
A layer of glass or transparent plastic is often used to protect the solar cell from environmental damage, such as moisture and debris. This cover also provides mechanical stability.
Materials Used in Solar Cells
Solar cells are typically made from a semiconductor materials. The most common materials used in the construction of solar cells are:
1. Crystalline Silicon (c-Si):
Crystalline silicon is the most widely used material in solar cells, accounting for about 90% of the market. It exists in two forms: monocrystalline and polycrystalline. Monocrystalline cells are more efficient but more expensive to produce than polycrystalline cells.
2. Thin-Film Materials:
These include amorphous silicon (a-Si), cadmium telluride (CdTe), and copper indium gallium selenide (CIGS). Thin-film solar cells are less efficient than crystalline silicon cells but are cheaper and can be applied to flexible surfaces.
3. Perovskite Materials:
Perovskite solar cells are a newer development and are gaining attention due to their high efficiency and low production costs. However, they are still in the research and development phase and are not yet widely used.
4. Organic Materials:
Organic photovoltaic cells use organic compounds (carbon-based) to convert sunlight into electricity. These materials are flexible, lightweight, and can be manufactured at low cost, though their efficiency is generally lower than that of inorganic materials.
Types of Solar Cells
There are several types of solar cells, each with its advantages and applications. The most of common types include:
1. Monocrystalline Solar Cells:
These cells are a made from a single, continuous crystal structure. They are the most efficient type of solar cell but also the most expensive. Monocrystalline cells are easily recognizable by their uniform, black appearance.
2. Polycrystalline Solar Cells:
Polycrystalline cells are made from silicon crystals that have been melted together. They are less efficient than monocrystalline cells but are cheaper to produce, making them a popular choice for residential solar installations.
3. Thin-Film Solar Cells:
Thin-film solar cells are made by depositing one or more thin layers of photovoltaic material onto a substrate. These cells are lightweight, flexible, and can be a manufactured at a lower cost. However, they have lower efficiency compared to crystalline silicon cells.
4. Perovskite Solar Cells:
Perovskite solar cells are a rapidly emerging technology, known for their potential to achieve high efficiency at a lower cost. They are made from a class of materials called perovskites, which can absorb light across a broad range of wavelengths.
5. Organic Solar Cells:
Organic solar cells are made from carbon-based materials and offer the promise of low-cost, flexible, and lightweight solar panels. However, they are still in the early stages of development and have lower efficiencies than traditional silicon cells.
Working Principle of Solar Cells
The working of a solar cell is based on the photovoltaic effect, first observed by French physicist Edmond Becquerel in 1839. The process can be a broken down into a few simple steps:

1. Absorption of Light:
When sunlight strikes the surface of a solar cell, photons from the light are absorbed by the semiconductor material (usually silicon). The energy from the photons excites electrons in the material, causing them to move from their regular atomic positions.
2. Creation of Electron-Hole Pairs:
The energy from the absorbed photons excites the electrons to move to a higher energy state, creating free electrons. At the same time, “holes” are created in the semiconductor structure. These are areas where electrons have vacated and can now accept other electrons.
3. Separation of Charges:
The electrons and holes are separated by an electric field that is created by the junction between the p-type and n-type materials in the cell. The n-type region contains excess electrons, while the p-type region has excess holes. This separation creates a voltage potential.
4. Movement of Electrons:
Once separated, the electrons move toward the n-type material, while the holes move toward the p-type material. This movement creates an electric current that flows through an external circuit, providing usable electricity.
5. Conversion to Direct Current (DC):
The flow of electrons generates a direct current (DC), which can then be used to power electrical devices or stored in batteries for later use.
Construction of Solar Cells
The construction of solar cells involves several layers, each performing a specific function in converting sunlight into electricity. The process of manufacturing a solar cell can be broken down into the following steps:
1. Wafer Production:
Solar cells start with the production of silicon wafers. Silicon, the most abundant material in the Earth’s crust, is purified and then cut into thin wafers. Monocrystalline wafers are made from a single crystal structure, while polycrystalline wafers are made from multiple crystal structures.
2. Doping:
To create the necessary electric field within the solar cell, the silicon wafer is “doped” with other materials. Doping adds impurities to the silicon, creating two regions: the n-type and p-type regions. The n-type region has an excess of electrons, while the p-type region has an excess of holes.
3. Application of Metal Contacts:
Once the wafer is doped, metal contacts are added to the top and bottom of the cell. These contacts allow the current generated by the cell to flow out to an external circuit.
4. Application of an Anti-Reflective Coating:
An anti-reflective coating is applied to the surface of the cell to reduce the amount of light that is reflected away from the cell. This increases the efficiency of the cell by ensuring more light is absorbed.
5. Encapsulation:
The solar cell is then encapsulated in a protective material, such as glass or plastic, to protect it from environmental damage.
Efficiency of Solar Cells
The efficiency of a solar cell refers to the amount of sunlight that is converted into usable electricity. The efficiency of a solar cell depends on several factors, including the materials used, the design of the cell, and environmental conditions such as temperature and shading.
1. Theoretical Efficiency Limit:
The maximum theoretical efficiency of a single-junction solar cell is about 33.7%, known as the Shockley-Queisser limit. This is because a significant portion of the sunlight that strikes the cell is either reflected, absorbed as heat, or passes through without being absorbed.
2. Actual Efficiency:
In practice, most commercial solar cells have an efficiency of around 15-22%. Monocrystalline cells tend to be the most efficient, followed by polycrystalline and thin-film cells.
3. Factors Affecting Efficiency:
- Temperature: Solar cells become less efficient as they get hotter. This is why it’s important to ensure proper ventilation and cooling mechanisms.
- Light Intensity: The efficiency of a solar cell increases with the intensity of sunlight, though it plateaus at a certain point.
- The angle of Incidence: The angle at which sunlight hits the solar panel affects the amount of energy absorbed. Optimal panel tilt is necessary for maximum efficiency.
Applications of Solar Cells
Solar cells are used in a wide variety of applications, including:
1. Residential and Commercial Solar Power Systems:
Solar panels are installed on rooftops to generate electricity for homes and businesses, reducing reliance on the grid and lowering energy bills.
2. Solar Farms:
Large-scale solar farms consist of thousands of solar panels arranged in arrays to generate electricity on a commercial scale. These farms can feed power directly into the electrical grid.
3. Off-Grid Applications:
Solar cells can power remote locations where access to electricity is limited or unavailable. This includes applications such as rural electrification, emergency power supplies, and powering remote telecommunication systems.
4. Consumer Electronics:
Solar cells are used in portable electronics such as calculators, watches, and phone chargers. These devices can be powered by small photovoltaic cells that generate electricity from ambient light.
5. Spacecraft and Satellites:
Solar panels are used to power spacecraft and satellites, where access to other energy sources is impossible.
6. Transportation:
Solar energy is being increasingly integrated into electric vehicles (EVs) and hybrid systems to extend their range and reduce dependence on fossil fuels.
Advantages and Disadvantages of Solar Cells
Advantages:
- Renewable Energy Source: Solar energy is inexhaustible and available in most parts of the world.
- Environmentally Friendly: Solar power does not emit greenhouse gases or pollutants.
- Low Operating Costs: Once installed, solar panels require minimal maintenance and have no fuel costs.
- Energy Independence: Solar energy allows users to become less reliant on grid electricity and fossil fuels.
- Scalability: Solar energy systems can be scaled to meet individual needs, from small residential systems to large commercial installations.
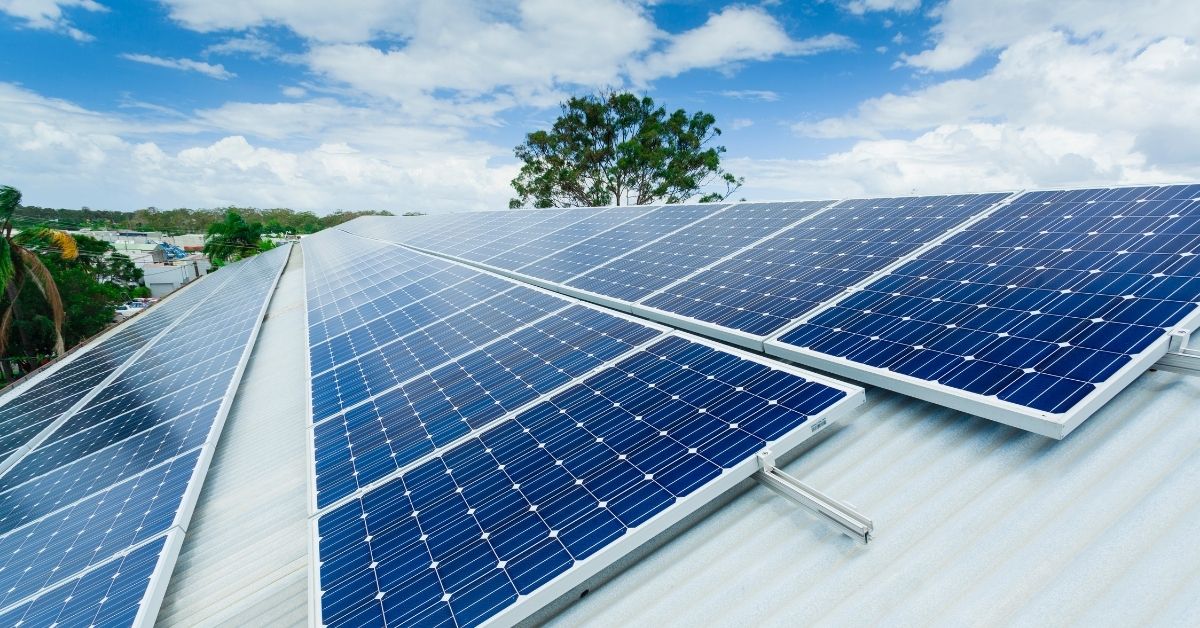
Disadvantages:
- High Initial Cost: The installation of solar panels requires a significant upfront investment.
- Intermittency: Solar power is dependent on sunlight, so energy production can fluctuate with weather conditions and time of day.
- Space Requirements: Solar panels require a large surface area to generate significant amounts of electricity.
- Energy Storage Costs: Storing solar energy for use at night or during cloudy weather requires expensive battery systems.
Future Trends and Advancements
The future of solar cells looks promising with ongoing research and development aimed at improving efficiency, reducing costs, and expanding applications. Some emerging trends and advancements include:
1. Perovskite Solar Cells:
These cells have the potential to surpass traditional silicon-based cells in efficiency while being cheaper to manufacture.
2. Bifacial Solar Panels:
Bifacial solar panels capture sunlight on both sides, increasing energy output without significantly increasing costs.
3. Solar Windows and Building-Integrated Photovoltaics (BIPV):
Solar cells can be integrated into building materials, such as windows and walls, to generate electricity while serving a dual purpose.
4. Solar Energy Storage:
Advancements in battery technology, such as lithium-ion and solid-state batteries, are making it easier to store solar energy for use during non-sunny periods.
5. Flexible and Lightweight Solar Cells:
The development of flexible solar cells opens up new possibilities for wearable technology and portable solar power solutions.
FAQs About Construction and Working of Solar Cells
Q1. What materials are solar cells made from?
Solar cells are primarily made from silicon, a semiconductor. Other materials may include cadmium telluride (CdTe), copper indium gallium selenide (CIGS), and organic photovoltaic materials, each offering different efficiencies and manufacturing processes.
Q2. How do solar cells convert sunlight into electricity?
Solar cells convert sunlight into electricity through the photovoltaic effect. When sunlight strikes the solar cell, photons are absorbed, knocking electrons loose from their atoms and creating a flow of electricity.
Q3. What is the role of the p-n junction in solar cells?
The p-n junction is a crucial component that creates an electric field. It is formed by combining p-type (positively charged) and n-type (negatively charged) semiconductors. This electric field helps separate and direct the flow of electrons and holes, generating electric current.
Q4. What factors affect the efficiency of solar cells?
Several factors influence solar cell efficiency, including the quality of the semiconductor material, the design of the cell, temperature, shading, and the angle of sunlight. Advanced technologies, such as bifacial cells and multi-junction cells, can also enhance efficiency.
Q5. What is the difference between monocrystalline and polycrystalline solar cells?
Monocrystalline solar cells are made from a single crystal structure, which typically results in higher efficiency and a longer lifespan. Polycrystalline cells, made from multiple crystal fragments, are generally less efficient but are often cheaper to produce.
Q6. How are solar cells manufactured?
The manufacturing process involves several steps: purifying silicon, forming it into wafers, doping the wafers to create the p-n junction, applying anti-reflective coatings, and assembling the cells into modules. Automation and precision are crucial throughout the process.
Q7. Can solar cells work in cloudy conditions?
Yes, solar cells can generate electricity even on cloudy days, though their efficiency is reduced. Solar cells can still capture diffuse sunlight, but overall energy output is typically lower compared to sunny conditions.
Q8. What advancements are being made in solar cell technology?
Ongoing advancements include the development of perovskite solar cells, which offer potential for higher efficiency and lower production costs. Researchers are also exploring bifacial cells, flexible solar panels, and solar cells integrated into building materials.
Q9. What is the lifespan of solar cells?
Most solar panels have a lifespan of 25 to 30 years, during which they gradually lose efficiency. Many manufacturers offer warranties for 25 years, ensuring that panels produce a certain percentage of their original capacity.
Q10. How do environmental conditions impact solar cell performance?
Factors such as temperature, humidity, and dust can affect solar cell performance. High temperatures can reduce efficiency, while dust accumulation can block sunlight. Regular cleaning and optimal installation can help mitigate these issues.
Solar cells are an essential technology in the transition to a more sustainable and renewable energy future. From the basic construction using semiconducting materials to the sophisticated mechanisms that allow the conversion of sunlight into electricity, solar cells represent a powerful tool in the fight against climate change and energy scarcity. As technology continues to evolve, the efficiency, affordability, and versatility of solar cells are expected to improve, making them a key component of global energy strategies.
Click here to learn more about Construction and Working of Solar Cells
Click here to learn more about How to Invest in Solar Projects